Ammonia as Key Enabler of the Energy Transition and Economic Equalizer
Shifting to renewable energies requires a fundamental change in the energy and chemical industries. Batteries will play a key role in short term storage, while chemical vectors (e.g. hydrogen, methane, methanol, ammonia) will be essential for seasonal storage and distribution. The chemical industry will have to (1) decarbonize heat generation by shifting to electrical heating or electrochemical technologies or sustainable fuels (e.g. hydrogen or biomethane), and (2) replace fossil feedstocks with more sustainable alternatives (biomethane, biomass, and plastic waste). For the utility companies the challenge will come from the integration of fluctuating renewables and chemical energy vectors with the electricity grid, which have different response rates.
Dr. Jimmy A. Faria
Dr. Ir. Faria is an Associate Professor in the Faculty of Science and Technology at the University of Twente. His research group develops smart catalysts and intensified processes for sustainable production of fuels and chemicals in close collaboration with industrial partners. He has recently received the Vidi grant for the valorization of plastic waste.
Chemical energy storage
Direct storage of hydrogen is complex and expensive. In fact, NH3 storage is more economically attractive than hydrogen already after one day. As a result, hydrogen conversion into ammonia, at the moment, is key to lower the levelized cost of electricity (LCOE) when dealing with inherently fluctuating renewables. Among all the power-to-chemicals storing strategies, ammonia offers a carbon-free pathway that can be economically feasible in large scales (MWh to TWh) using scalable technologies (Figure 1) [1].
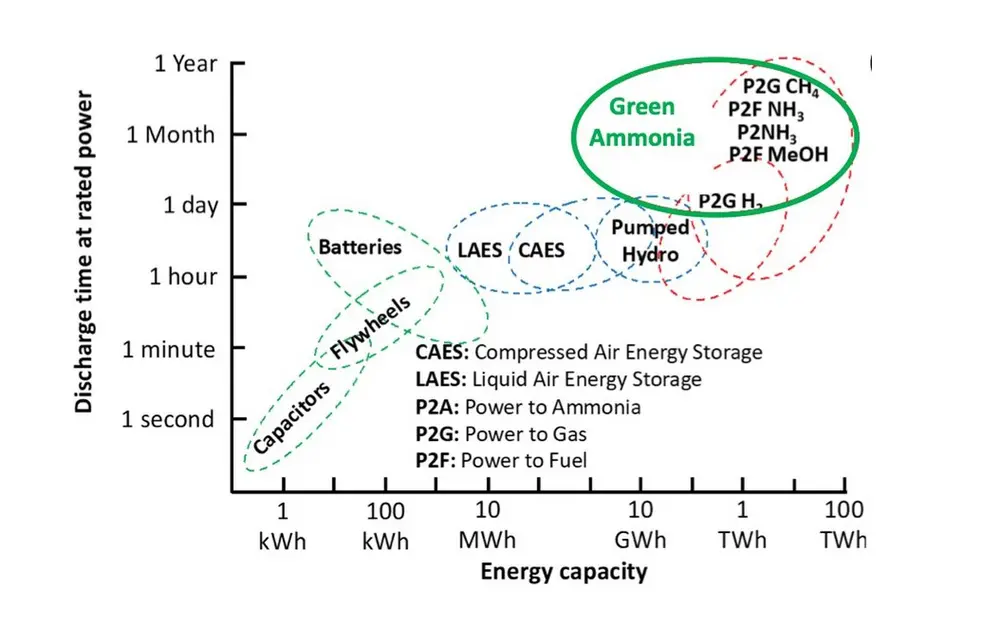
Figure 1: Qualitative capacity and discharge rate for storage of electricity for different technologies (adapted from reference [1])
Green Ammonia
Ammonia Haber-Bosch synthesis is perhaps the most transformative chemical processes in modern human history. In this process nitrogen and hydrogen react on a Fe-based catalyst to produce ammonia at high temperatures (400–500 °C) and pressures (60–180 bar). This process allows the production of nitrogen-based fertilizers (e.g. urea and ammonium nitrate) in large scales at low costs, substantiating the population growth observed in the last century.
Unfortunately, the dependency of this process on fossil feedstocks leads to a significant C-footprint. As a result, the HB process is responsible for ca. 1.6% of the anthropogenic CO2 emissions worldwide. Reforming of biomethane and/or biomass via gasification, or electrochemical water splitting using renewable electricity are key to produce "green" ammonia. The recent decrease in renewable electricity costs and hydrogen production technologies (i.e. electrolyzers) has enabled the production of green hydrogen in large scales. The beauty of this pathway is that the advent of green hydrogen could potentially reduce the footprint of ammonia from 1.6 to ca. 0.1 tCO2/tHN3. In this picture, ammonia will play a pivotal role for either direct use as fuel in power generation or as hydrogen carrier for chemical synthesis (see Figure 2).
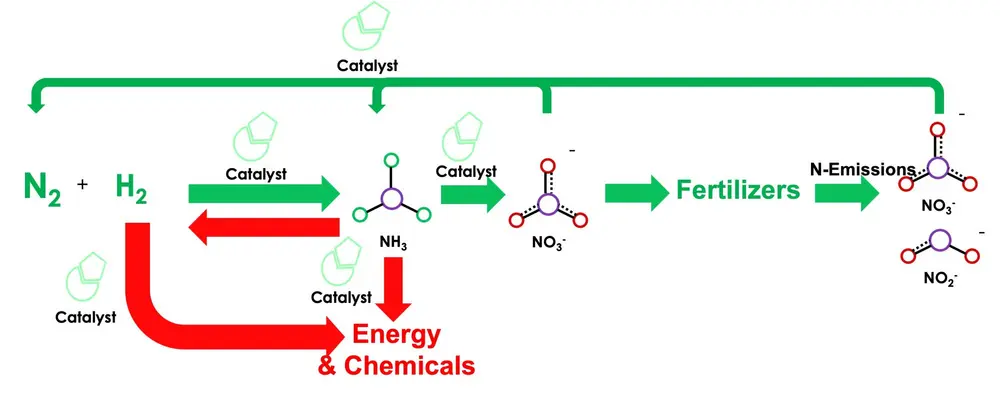
Figure 2: Ammonia synthesis as energy carrier (red) and chemical fertilizer (green)
Centralized vs. Distributed
The combination of electrochemical water splitting with HB ammonia synthesis process can lead to an energy footprint as low as ca. 8 kWh/kgNH3 at scales of ca. 105 kgNH3/h (Figure 3). Unfortunately, the energy consumption of the Haber-Bosch process increases to 22 kWh/kgNH3 at small scales (e.g. 0.5 kgNH3/h). This has become a major hurdle in the implementation of this technology in small countries and developing countries, like the Small Island Developing States (SIDS).
By lowering the cost of electricity, using green ammonia, it is possible to lower the final costs of seawater desalination
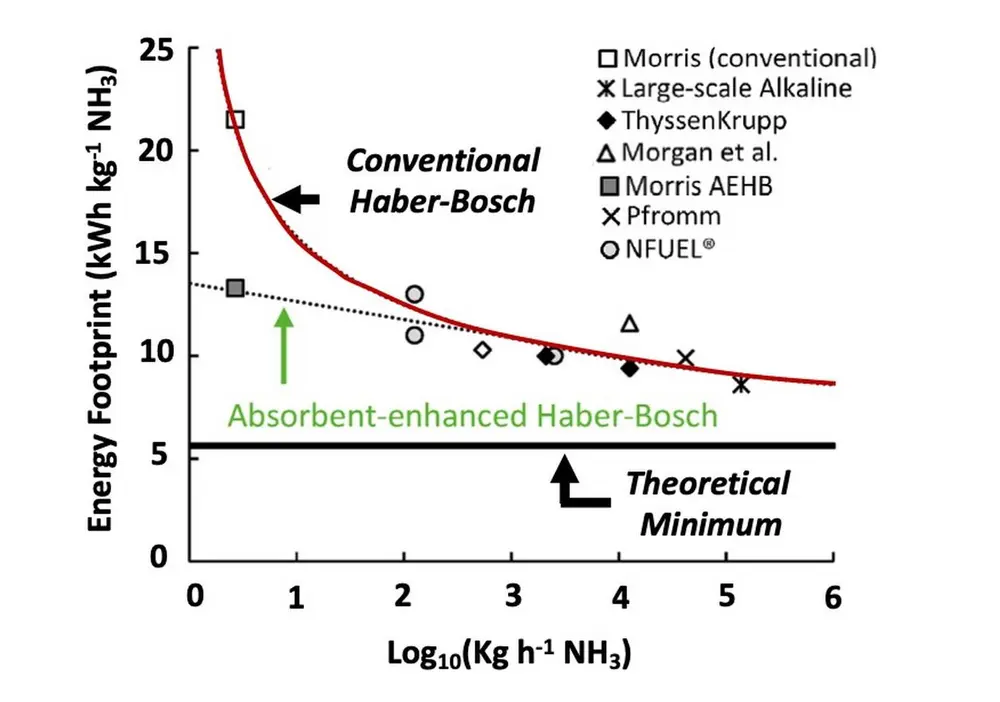
Figure 3: Energy footprint of electrolysis-based Haber-Bosch (adapted from reference [2])
Green ammonia production in SIDS can be a major asset as this chemical can link the water, energy, and food sectors (Figure 4). On one hand, ammonia has a high hydrogen content (17.65 wt. %), it can be easily stored and transported as a liquid at room temperature and ~ 10 bar of pressure, and it has higher energy density (12.7 MJ/L) than that of liquid hydrogen (8.5 MJ/L), making it a great energy carrier. This storage capacity can reduce the operational costs of the renewable energy plants, allowing cheaper electricity generation.
Considering that in SIDS the access to freshwater is limited, the utilization of energy-intensive desalination techniques, e.g. seawater reverse-osmosis, is often unavoidable. By lowering the cost of electricity, using green ammonia, it is possible to lower the final costs of seawater desalination. Similarly, ammonia production can facilitate ultimately fertilizer generation locally in SIDS, potentially reducing their dependence on food imports.
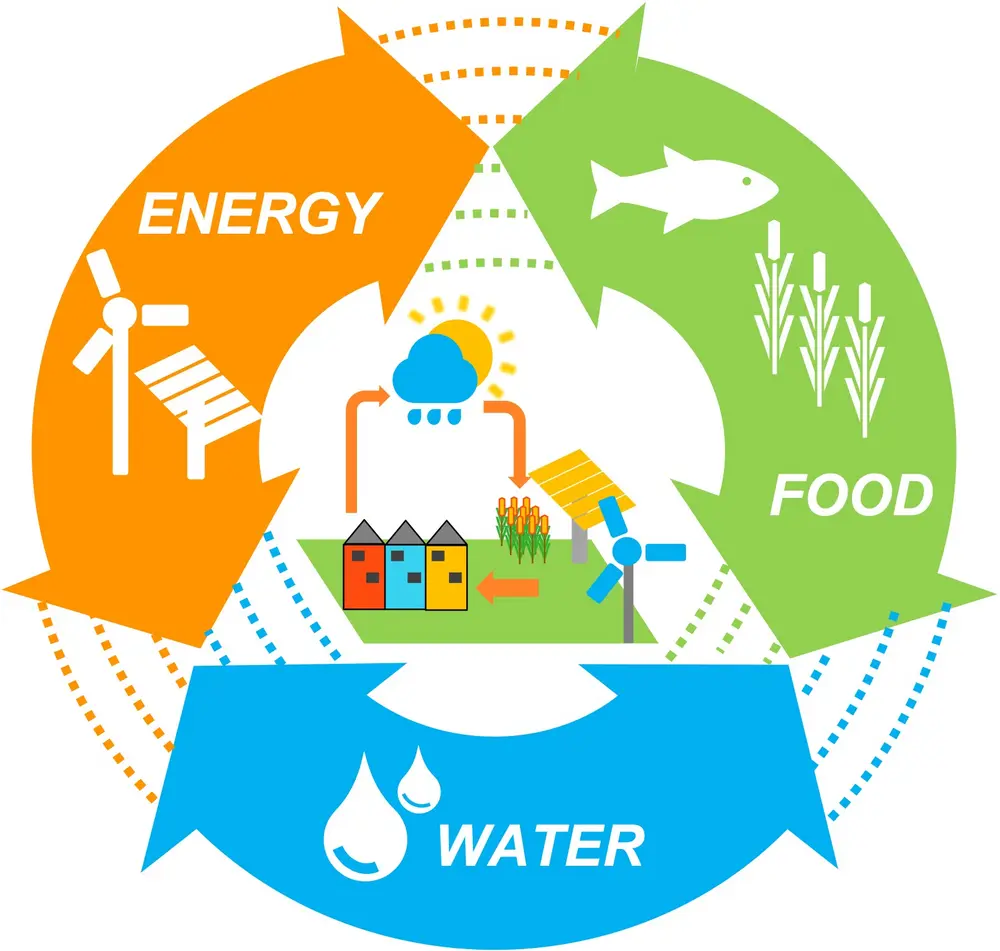
Figure 4: Water-Energy-Food Nexus enabled by green ammonia
For instance, in the island of Curaçao the average price of electricity is ca. 0.35 USD/kWh. This value is substantially higher than those in the US (0.10 USD/kWh) and Europe (0.22 USD/kWh). This is due to the elevated prices of imported fuels, which increases the cost of living and reduces the competitiveness of the goods produced locally. Despite having vast wind and solar resources the share of renewables in the electricity mix is typically low. Developing energy storage technologies that can enable larger shares of renewables are key for these regions.
To facilitate the conversion of ammonia in small scales at low operating pressures researchers at the University of Minnesota devised a process that uses an absorbent to remove the ammonia present in the product stream, enabling the separation of ammonia before recycling the unreacted feed. Thanks to this innovative separation, the process could operate at lower pressures (10–50 bar). This so-called 'absorbent enhanced Haber-Bosch or AEHB' is less energy intensive and cheaper to downsize.
Next to this development, the group led by Prof. H. Hosono at the Tokyo Institute of Technology has paved the way towards low-temperature ammonia synthesis using transition metals supported on electron donor and hydrogen storage materials so-called metal hydrides. Recently, they achieved catalytic activities of ca. 50 μmol*g-1*h-1 at temperatures as low as 50 °C and 0.1 MPa of pressure using a ruthenium-based catalysts (Ru/CaFH). These catalysts can outcompete any commercial catalyst in terms of activity. The main challenge that remains is the reliance on scarce noble metals and the demonstration of stable operation in the presence of impurities such as water and oxygen in the feed.
Developing materials that can absorb ammonia in large quantities with minimum deactivation is key
Leveraging the AEHB reactor concept and the highly active Ru-based catalysts, we developed a process concept to produce green ammonia that can provide on-demand electricity in islanded regions (power to ammonia to power or P2A2P as shown in Figure 5) [3]. In a nutshell, the system consisted of a windfarm coupled to a zero liquid discharge seawater desalination plant and water electrolysis/battery system, a so-called Battolyzer, which could either generate electricity for direct use or produce hydrogen. The hydrogen generated was employed to make green ammonia. This ammonia was stored for later use in the months with lower wind-power generation. The energy content of the ammonia was transformed into electricity using Ni-based Solid Oxide Fuel Cells (SOFCs) that can produce electricity via ammonia oxidation.
We investigated the impact of this approach for the island of Curaçao as archetypical of the Small Island Developing States (SIDS). Our model indicates that the levelized cost of electricity (LCOE) for the combined wind and ammonia energy storage system can be as low as 0.13 USD/kWh, making this carbon-free alternative competitive with the conventional power plants operating with heavy fuel oil (0.15–0.17 USD/kWh).
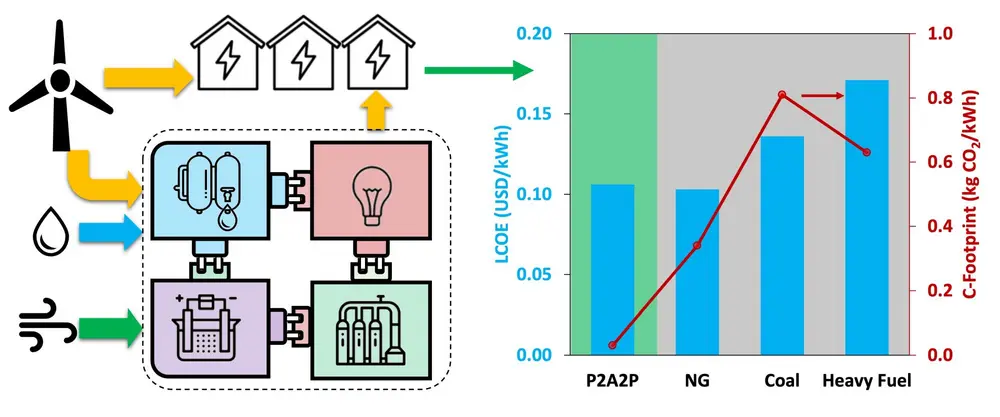
Figure 5: P2A2P concept benchmark against NG, gas, and heavy fuel
More recently, we revised this model and pondered the impact of green ammonia imports in the costs of renewable electricity in the islands of Curaçao and Viti Levu in Fiji, which are representative SIDS with high and low availability of wind energy, respectively [4]. In the absence of ammonia imports, the LCOE in Viti Levu was rather high (1.10 USD/kWh) in comparison to Curaçao (0.12 USD/kWh) due to the low utilization factors of the windfarm.
Notably, for Viti Levu transitioning to fully renewable energy leveraging green ammonia imports reduces the LCEO to 0.35–0.42 USD/kWh (ammonia import prices of 400–800 USD/ton). In contrast, for Curaçao the LCOE varied from 0.10–0.15 USD/kWh for the same ammonia costs bracket.
Scientific and Engineering Gaps
For small scale operations, the main challenges are in the development of stable and highly active ammonia synthesis catalysts based on earth abundant elements. The Ru-based catalysts supported on metal hydrides are extremely sensitive to water and oxygen, which hinders industrial scale-up. Unfortunately, by increasing the activity of the catalyst towards nitrogen-nitrogen bond dissociation, one enhances the reactivity of the surface to other species, which might be present in the feedstock. Reducing the concentration of these impurities is economically prohibiting, thus developing oxygen- and water-resistant catalysts is a major priority.
Likewise, for the AEHB process improving stability is key. The metal halide absorbents undergo drastic changes in volume, which leads to mechanical breakup of the solids and particle growth via sintering during cycling. Thus, developing materials that can absorb ammonia in large quantities with minimum deactivation is key.
In terms of engineering, it is essential to improve further the energy efficiency of electrolyzers
Next to the ammonia synthesis, one might be interested in recovering pure hydrogen. In this case, catalytic materials that can crack ammonia back to hydrogen at low temperature are essential, as commercial Ni-based reforming catalysis operates at 700–900 °C. Next, achieving high purity will be crucial for facile integration in existing NG pipelines.
In terms of engineering, it is essential to improve further the energy efficiency of electrolyzers. This step is responsible for more than 60% of the total energy consumption of the power to ammonia system (i.e. 8.7 kWh/kg NH3 out of the 13.9 kWh/kg NH3). This value is higher than those often found in ammonia synthesis from NG (7.8 kWh/kg NH3). Thus, finding more efficient processes for hydrogen production will be central for the widespread implementation of green ammonia.
Finally, the potential environmental impact of large-scale deployment of the hydrogen economy leveraging green ammonia must be carefully considered. Here, one could wonder how water electrolysis, which requires high-purity water, could impact freshwater stocks. Likewise, the potential impact of increasing the production capacity of ammonia by a factor of three to four to cope with increased demands as hydrogen carrier, shipping fuel, and power generation must be central in the assessment of future green ammonia projects.
REFERENCES
[1] ISPT, Power to Ammonia, Report. (2017). http://www.ispt.eu/media/ISPT-P2A-Final-Report.pdf (accessed February 5, 2021).
[2] K.H.R. Rouwenhorst, A.G.J. Van der Ham, G. Mul, S.R.A. Kersten, Islanded Ammonia Power Systems: Technology Review & Conceptual Process Design, Renew. Sustain. Energy Rev. 114 (2019). https://doi.org/10.1016/j.rser.2019.109339.
[3] V.N. Sagel, K.H.R. Rouwenhorst, J.A. Faria, Green Ammonia Enables Sustainable Energy Production in Small Island Developing States: A Case Study on the Island of Curaçao, Renew. Sustain. Energy Rev. 161 (2022) 112381. https://doi.org/10.1016/j.rser.2022.112381.
[4] V.N. Sagel, K.H.R. Rouwenhorst, J.A. Faria, Renewable Electricity Generation in Small Island Developing States: The Effect of Importing Ammonia, Energies. 15 (2022) 3374. https://doi.org/10.3390/en15093374.